main content A Quantum Leap for Physics Students
Ben Olsen, assistant professor of physics, is establishing Lewis & Clark’s first Quantum Information Science and Engineering lab to probe how unusual types of matter behave at the subatomic level. But first he and his students have to build “The Apparatus.”
Groundbreaking Science







The steel structure occupying one end of a long table in Ben Olsen’s lab consists of two tower-like structures and a glass-walled chamber made of titanium. The device could easily be mistaken for the model of an interstellar space station in a Star Wars film. In actuality, its purpose is both much smaller and much grander than that.
When it’s done, “The Apparatus”―as Olsen and his students call it―will be the only device like it in the state of Oregon, custom built for quantum simulation experiments. Olsen, assistant professor of physics, will use it to study the behavior of materials at small scales. The collection of particles they will be studying is about 0.0001 meters across, roughly the thickness of human hair. At such small scales, materials’ behavior can be unexpected, even counter to the conventional laws of physics.
“The behavior we are studying is essentially the macroscopic behavior you can’t predict based on microscopic interactions,” he says. “One way to understand it is to imagine a little pond. The lithium atoms are waves on the pond. They pass through each other and interact with each other.”
Olsen’s research is focused on “many body” quantum systems, which are made up of a number of different interacting waves. The experiments he is planning on ultracold lithium atoms will illuminate the emergent quantum behavior of such systems.
“We have talented scientific minds here at Lewis & Clark who think about things really differently than I do. In the long term, I would like for their ideas to contribute to the direction of the research.”
–Ben Olsen, assistant professor of physics
“Instead of particles bouncing off each other, when atoms are behaving quantumly, they’re behaving like waves. And that’s the realm of quantum mechanics: where everything is a wave,” says Emma Hataway BA ’25, a physics and mathematics double major who has been working in the lab for almost a year and a half. Hataway’s work focuses on building and characterizing the lab’s permanent magnets and electromagnets.
This year, Olsen’s additional student researchers include Emma Falk BA ’26, Morgan Berghof BA ’26, and Kaia O’Neill BA ’26, all of whom are physics and mathematics double majors, planning to pursue further studies after graduation. They credit Olsen’s mentorship for helping to shape their interest in quantum research.
It is the interactions between the waves that determine the material’s behavior, which makes data collection and analysis particularly challenging. Understanding and learning to control the behavior of these quantum systems is crucial for developing next-generation technologies used for imaging, sensing, computing, modeling, and communication.
“My favorite specific technology this work could be relevant for are superconductors, which are materials that transmit electricity with no heating and, therefore, using less energy,” says Olsen. “Anything that uses electricity would benefit from superconducting technology since less battery usage means less wasted power.”
It can also help explain little understood natural phenomena like neutron stars, which are formed when a massive star runs out of fuel and collapses, forcing protons and electrons into the core to form a neutron.
“When they collapse, everything but the neutrons get pushed out to the surface, and in the core, all that’s left are the neutrons. Neutron stars are the densest matter in the universe. The only way to figure out what a neutron star is doing is to simulate it like this,” says Olsen, who earned his PhD at Princeton University and joined the Lewis & Clark faculty in 2023.
“Astronomers can make observations of neutron stars, but they’re really far away, and we can’t do anything to change their environment,” says Olsen. “The only way to see how they respond to changes is through simulations like the ones we make in the lab.”
With this work, Olsen is establishing the first Quantum Information Science and Engineering (QISE) lab at Lewis & Clark. He was recently awarded a grant from the National Science Foundation for his project titled, “LEAPS-MPS: Ultracold Atoms for Quantum Science.” The project is supported by NSF’s Launching Early-Career Academic Pathways in Mathematical and Physical Sciences (LEAPS-MPS) program.
In addition to enabling Olsen to acquire the equipment to build “The Apparatus,” the NSF grant also supports his undergraduate research students over the course of two years, during which time they will have the opportunity to participate in full-time paid summer research experiences through the Rogers Science Research Program.
“I have my own ideas for what work I’d like to do, but my hope is that eventually my students will help decide what we do each week,” says Olsen. “We have talented scientific minds here at Lewis & Clark who think about things really differently than I do. In the long term, I would like for their ideas to contribute to the direction of the research.”
Credit: Nina Johnson
Creating Quantum Gases, the “Coolest” Material in the Universe
Olsen and his students will use powerful magnets to trap lithium atoms and then bombard them with lasers in order to cool them down to a really low temperature. It is this action that brings out the atom’s quantum behavior.
“We are going to trap the atoms in a pencil-thin line right there,” says Olsen, referring to the glass-walled chamber, “and then a laser beam comes in to nudge them further.”
Olsen offers what he calls “a cartoon way” to think about the process. “When an atom is hot, it’s moving around a lot. If it starts moving toward one laser beam, another laser beam pushes it back. Add more laser beams from different directions, and each one pushes the atom back toward the center.”
Using six laser beams—pointing in opposite directions along three axes—“any motion away from the center is going to get pushed on by a laser,” Olsen explains, “until the material collects in a cold ball.”
“This process describes one phase of cooling that gets the atoms to cold, .001 Kelvin, but not ultracold, .0000001 Kelvin,” says Olsen. The lowest temperature possible is 0 Kelvin. “We’ll need another stage of evaporative cooling in an optical trap―just using focused laser light to hold the atoms in place―in order to get to the quantum regime.”
At that point, the lithium atoms are colder than just about anything in the known universe, just a few billionths of a degree above 0 Kelvin or absolute zero. This is equivalent to -459.67 degrees Fahrenheit.
Readying “The Apparatus” for Quantum Simulations
Credit: Nina Johnson
The team aims to start the first experiments in summer 2026. Meanwhile, they are building and preparing “The Apparatus.”
“I do a lot of the computer simulations of the magnets and coding,” says Falk, who has been working in the lab for the past year and a half.
“I have also been looking at the construction of the magnets and tolerancing,” she adds, referring to her work studying how the coils generate magnetic fields and waste heat. Falk simulates how slight errors in the spacing or alignment might degrade the magnetic field.
Other preparatory tasks include silver plating all of the stainless steel screws used in construction so they don’t doesn’t fuse with the stainless steel of the device when heated. They also recently finished “baking” the parts of the device in a very high-temperature oven in order to “cook out” the hydrogen so it doesn’t leak into the chamber with lithium atoms.
When the experiments begin, Olsen hopes that their quantum simulations will help illuminate the atomic structures that underlie matter. These simulations can help model the behavior of materials made up of crystalline lattices, the building blocks for many types of familiar matter, such as metals and ceramics. Understanding them is essential for predicting and designing materials with special qualities such as the ability to conduct heat, electrical, or optical properties which could be used for solar cells and laser imaging.
“Some of the quantum simulations will mimic crystalline lattices, but some will mimic other materials,” said Olsen. “We can adjust our lasers to mimic 3D, 2D, and 1D systems: blobs, pancakes, or lines.”
The goal of running these experiments, says Olsen, is to contribute to a guide, or a “cookbook” for making these structures.
Adds Olsen, “Our work can tell researchers: ‘If your crystal does this, then it’s not going to do what you want it to do, but if it does this, then it might.’ This could lead to all sorts of breakthroughs in the development of materials prized for qualities like magnetism and superconductivity. And, it will tell us more about the universe we live in.”
More Stories

Textual Treasures
Paging Through the Past
In her Medieval Manuscripts course, Professor of English Karen Gross brings history to life with the help of Watzek Library’s rich archival collections. Students get hands-on experience with centuries-old texts as they explore the art of archival research.

Dance Moves
NYC-Based Dance Company In Step With L&C Students
The Tiffany Mills Dance Company, named for and headed by L&C’s director of dance, took part in a spring residency on campus, leading a series of community dance workshops and performing The Viola Trilogy alongside students.
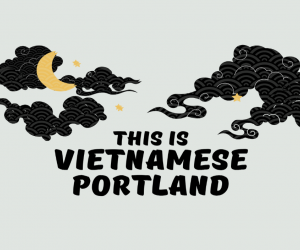
Immersive Learning
Voices of Vietnamese Portland
Nhân Hàn BA ’27 and Thoan Nguyễn BA ’27, with project manager Zoë Maughan BA ’19, curated a 15-panel, bilingual traveling exhibit highlighting stories from Vietnamese Portland: Memory, History, Community, an archive documenting experiences of Vietnamese Portlanders.
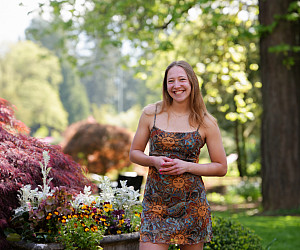
Graduation Countdown
Senior Speaker: Katie Ingersoll BA ’25
Katie Ingersoll BA ’25 will address graduates at the College of Arts and Sciences commencement on May 10.